Neuroscience Research Products and Resources
Neuroscience research requires a multidisciplinary approach, integrating concepts and experimental approaches from physiology, biochemistry, cellular and molecular biology, computational biology, and behavioral biology to elucidate the intricate cellular networks controlling behaviors.
Contents
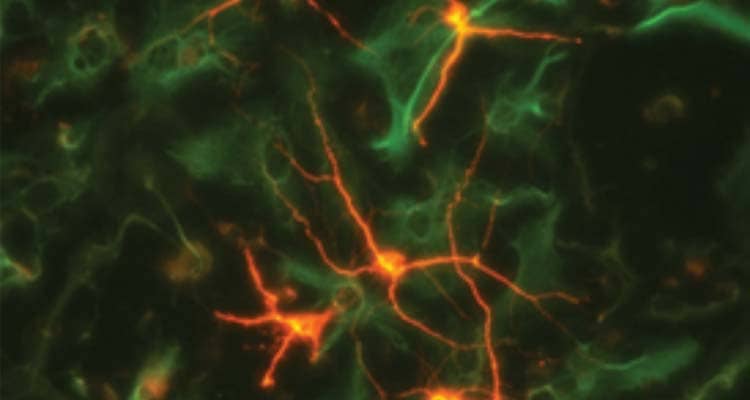
Neuroscience Disease Types
Browse research products by Neuroscience Disease Type:
Alzheimer's Disease | Amyotrophic Lateral Sclerosis | Depression | Epilepsy |
Huntington's Disease | Multiple Sclerosis | Pain | Parkinson's Disease |
Schizophrenia |
Neuroscience Research Areas
Browse research products by Neuroscience Related Research Area:
Neuroscience Targets
Browse research products by Neuroscience Target:
Visualize Multiple Markers Simultaneously in Tissue
Detect characterize and localize mRNA in the nervous system with RNAscope® ISH.
Neuroscience Research Resources and Tools
View All Tools and Resources
- Spectra Viewer
- Simple Plex Panel Builder
- Simple Western Assay Kit Builder
- Simple Western Antibody Database
- Milo Antibody Database
- Flow Cytometry builder
Neuroscience Background Information
Two major areas of neuroscience research, Molecular and Cellular neuroscience and Developmental neuroscience, have provided the foundation for understanding brain disease mechanisms and developing therapeutic approaches for a wide range of conditions including Neurodegenerative and Neuroinflammatory diseases. Neurodegenerative diseases, or conditions leading to the irreversible loss of nerves and concomitant loss of function, impact the health of millions of people around the globe. Among these, Alzheimer’s and Parkinson’s disease constitute the leading cause of dementia worldwide.
Molecular and Cellular Neuroscience
Molecular and Cellular Neuroscience focuses on the molecules and genes controlling brain cellular function.
Synaptic Transmission
Neurotransmission, or synaptic transmission, refers to the passage of signals from one neuron to another, allowing the spread of information via the propagation of action potentials. This process is the basis of communication between neurons within, and between, the peripheral and central nervous systems, and is vital for memory and cognition, muscle contraction and co-ordination of organ function.
The majority of neurotransmission occurs across chemical synapses, where an endogenous neurotransmitter is released by the presynaptic neuron and detected by receptors on the postsynaptic neuron. Neurotransmitters can be broadly split into three categories; amino acids including glutamate and glycine, amines including dopamine (DA), serotonin (5-HT; ) and norepinephrine (NE), and peptides such as dynorphin, the enkephalins and neuropeptide Y.
While the amino acids glutamate and glycine are found in all cells of the body, other neurotransmitters are only synthesized by neurons. Following synthesis, neurotransmitters are taken up and stored in synaptic vesicles, ready for release. The release of a neurotransmitter is triggered by the arrival of action potentials in the axon terminal of the presynaptic neuron, opening voltage-gated Ca2+ channels and allowing influx of ions. The resulting elevation in intracellular Ca2+ concentration causes synaptic vesicles to merge with the presynaptic membrane, releasing the neurotransmitter into the synaptic cleft by exocytosis.
Neurotransmitters cross the synaptic cleft and bind to their specific receptors. These maybe ligand-gated ion channels (LGICs) or G protein-coupled receptors (GPCRs), with some neurotransmitters having receptors in both categories. Binding of a neurotransmitter to a LGIC causes a conformational change in the structure of the protein, allowing the passage of ions through the channel. Passage of ions through channels that are selective for positively-charged cations results in depolarization of the postsynaptic membrane and initiation of an action potential in the postsynaptic neuron. In contrast, passage of ions through negatively-charged, anion selective channels results in hyperpolarization of the postsynaptic membrane, so inhibiting action potential initiation. Binding of a neurotransmitter to a GPCR results in the activation of G proteins, which are then able to act on enzymes to modulate intracellular signaling pathways. The end result of this is the modulation of activity of other proteins, including ion channels and enzymes.
Once a neurotransmitter has bound to its receptor, it is cleared from the synaptic cleft to allow another wave of synaptic transmission. Neurotransmitter molecules are taken up by the presynaptic neuron, or by other cell types such as astrocytes, via specific reuptake transporters. Neurotransmitters may then be metabolized to be reused for further production, or they can be recycled into synaptic vesicles.

This above schematic shows the main events during dopaminergic, glutamatergic, opioid peptide and serotonergic neurotransmission. DA and 5-HT are both biogenic amines that are derived from amino acids, while glutamate itself is an amino acid and opioid peptides are cleaved from precursor proteins. All neurotransmitters undergo exocytosis from the presynaptic membrane and cross the synaptic cleft where they bind to their specific receptors. These receptors may be ligand-gated ion channels, such as ionotropic glutamate receptors, or G protein-coupled receptors, such as all subtypes of opioid receptor. Passage of ions through a ligand gated ion channel alters the excitability of a neuron. The action of neurotransmitters at GPCRs alters intracellular signaling pathways, with the specific pathway being dependent on the G protein-coupled to the receptor.
Action Potentials
An action potential is the signal that conveys information along a neuron and is also the trigger for release of a neurotransmitter at a synapse. Physically, an action potential is the rapid reversal of the resting membrane potential, caused by opening and closing of voltage-gated ion channels. At rest, the cytosol of a neuron is negatively charged (polarized) with respect to extracellular fluid, due to the distribution of ions across the cell membrane./p>
An action potential is initiated by the opening of voltage-gated Na+ channels (Nav channels) allowing influx of Na+ down its concentration gradient. This depolarizes the cell membrane past the threshold for action potential initiation. As Nav channels become inactivated, preventing the flow of Na+, voltage-gated potassium channels (Kv channels) open allowing the efflux of K+. This causes repolarization of the cell membrane, as the balance of ion movement across the membrane leads to the cytosol being more negatively charged than extracellular fluid. When Kv channels are open the cell membrane is highly permeable to K+, but permeability to Na+ is low as Nav channels are still inactivated. This leads to a period of hyperpolarization until Kv channel close, and the resting membrane potential is re-established.
Propagation of an action potential occurs as a wave of depolarization that spreads along an axon. When an area of the membrane becomes depolarized, it opens neighboring Nav channels, which then allow depolarization of that section of the membrane. The inactivation of Nav channels in the preceding section of membrane ensures that an action potential travels in only one direction alone an axon. Some axons in the central nervous system have a sheath around them, composed of myelin, which acts as an electrical insulator. Nav and Kv channels are localized on gaps in the myelin sheath, known as nodes of Ranvier. Myelination increases that speed of action potential propagation as the action potential effectively ‘hops’ along an axon, occurring only at nodes of Ranvier in a process known as saltatory conduction.
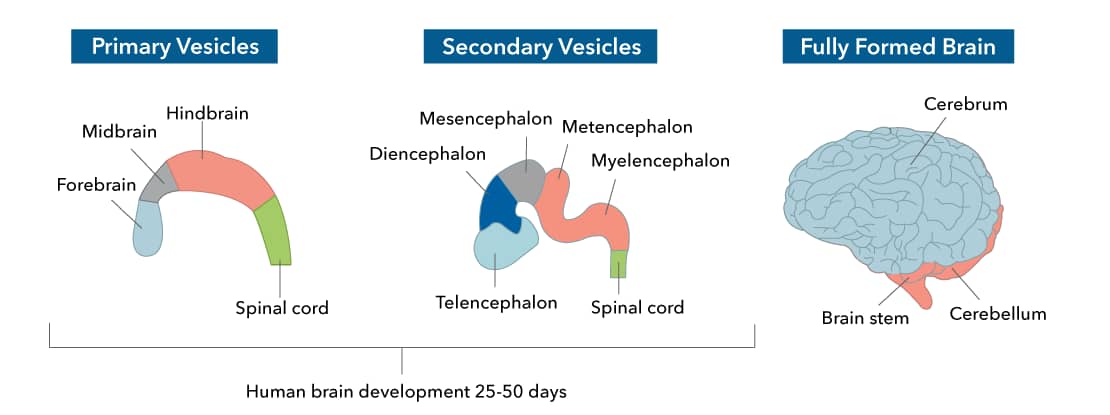